Protecting the esophagus from thermal injury during radiofrequency ablation with an esophageal cooling device.
Marcela Mercado Montoya11, Steven Mickelsen22, Brad Clark33, Martin Arnold4, Joseph Hanks5, Eric Sauter6, Erik Kulstad7
1
Bioengineering Department, Engineering Faculty, Universidad de Antioquia UdeA; Calle 70 No. 52-21, Medellín, Colombia.2
University of Iowa Carver College of Medicine, Department of Internal Medicine, 200 Hawkins Drive, Iowa City, Iowa 52242 .3
Riverside Medical Center, Department of Cardiology, 350 N Wall St, Kankakee, IL 60901 .4
University of Erlangen, Department of Cardiology, Ulmenweg 18, 91054 Erlangen, Germany.5
Riverside Medical Center, Research Department, 350 N Wall St, Kankakee, IL 60901 .6
A Scientific Venture, Inc., 18140 8th Ave. N, Plymouth, MN 55447 .7
Department of Emergency Medicine, UT Southwestern Medical Center, 5323 Harry Hines Blvd., MC 8579, Dallas ORCID..
We sought to quantify the capabilities of a commercially available cooling device to protect the esophagus from RF injury in an animal model and develop a mathematical model to describe the system and provide a framework from which to advance this technology.
A series of ablations (10 W, duration 30-45 seconds) were performed directly on exposed swine esophagus. Control ablations were performed with static 37°C water, and treatment ablations were performed with water (range 5°C-37°C) circulating within the device. Mucosal lesions were evaluated visually and with target tissue histology. A mathematical model was then developed and compared against the experimental data.
All 23 ablations (100%) performed under control conditions produced visible external esophageal lesions; 12 of these (52%) were transmural. Under treatment conditions, only 5 of 23 ablations (22%) produced visible external lesions; none (0%) were transmural. Transmurality of lesions decreased as circulating water temperature decreased, with absolute reduction ranging from 5.1% with the use of 37°C water (p=0.7) to 44.5% with the use of 5°C water (p<0.001). Comparison to the mathematical model showed an R^2 of 0.75, representing good agreement.
Under worst-case conditions, with RF energy applied directly to the adventitial side of the esophagus, internal esophageal cooling with an esophageal cooling device provides significant protective effect from thermal injury. A mathematical model of the process provides a means to further investigate this approach to preventing esophageal injury during RF ablation and can serve to guide ongoing clinical investigations currently in progress.
Key Words : atrial fibrillation, ablation, pulmonary vein isolation, esophageal protection, radiofrequency energy, esophageal cooling, finite element model, mathematical modeling.
Correspondence to: Marcela Mercado Montoya, University of Antioquia, Bioengineering Department, Calle 70 No. 52-21, Medellin, Colombia.
Ablation of the left atrium to achieve pulmonary vein isolation (PVI) is a standard therapy in the management of atrial fibrillation; however esophageal injury is a known potential consequence of this procedure [1,2]. Delivery of the radiofrequency (RF) energy necessary to perform PVI has the potential to cause injury to the nearby esophagus and its associated vagal innervation, with injuries including ulceration, hematoma, spasm, disorders of esophageal motility, and atrial-esophageal fistula (AEF), the latter representing the extreme consequence of esophageal thermal injury due to PVI.[1,2] Esophageal mucosal lesions are the likely precursor to AEF, and esophageal mucosal lesions are commonly found on endoscopy after PVI (with an incidence ranging from 3% to 60%).[3,4]
Luminal esophageal temperature (LET) monitoring is one proposed measure to reduce the incidence of esophageal injury during PVI; however, the success of temperature monitoring has varied widely [5,6] since effective measurement of LET depends on the position of the temperature probe relative to the heated cardiac tissue and also on good contact with the esophageal mucosa.[7] Moreover, the temperature probe used for esophageal temperature monitoring may contribute to a thermal effect and enhance direct tissue heating.[8] Recent data show rates of esophageal lesions in 40% to 50% of patients, regardless of whether a single-sensor or multi-sensor temperature probe is used.[3]
Esophageal injury prevention via cooling of the esophagus (primarily with various balloon configurations) has been investigated with mathematical modeling, preclinical models, and in the clinical arena, with an abundance of data showing efficacy in this approach. [9-16] The complexity of earlier prototypes, and the workflow disruption inherent in their use, appears to have precluded commercialization of a device leveraging this method. An esophageal heat transfer device (EnsoETM, Attune Medical, Chicago, IL, USA) is available for a variety of patient temperature management needs.[17-20] This device provides a closed-circuit of water flow through a multi-channel 12 mm diameter cylindrical silicone tube placed in the esophagus analogously to a standard orogastric tube, and warms or cools a patient through conductive heat transfer across the esophagus as well as convective heat transfer through the device.[17] The device is used for purposes such as the intentional reduction of patient body temperature below normal, the reduction of patient temperature from hyperthermic levels to normal range, and the prevention of inadvertent perioperative hypothermia. [17-20] Most patients treated with the device are endotracheally intubated; however, placement in sedated patients is also performed successfully. We aimed to quantify the protective effect of this device against thermal injury to esophageal mucosa in an animal model, and to develop a mathematical model that accurately describes the system, allowing further investigation into this approach to esophageal protection.
This pilot study was performed under protocol ACD001-IS75 approved by the Institutional Animal Care and Use Committee (IACUC) of American Preclinical Services, Minneapolis, MN. The study utilized methods consistent with current veterinary and USDA standards, with a state-of-the-art, Association for Assessment and Accreditation of Laboratory Animal Care (AAALAC) International-accredited vivarium. Animal care and handling was in accord with Office of Laboratory Animal Welfare guidance for humane care and use of animals and with regulations outlined in the USDA Animal Welfare Act (9 CFR Parts 1, 2 and 3) and conformed to the conditions specified in the Guide for the Care and Use of Laboratory Animals (National Academy Press, Washington DC, 1996). A swine model was selected due to similarities in size, physiology, and thoracic anatomy to typical adult human subjects undergoing PVI for the prevention of atrial fibrillation.
A total of six male Yorkshire swine weighing a mean of 81.5 ± 7 kg, housed on site, were given 12 hours food restriction but free access to water before the intervention. Subjects were medicated with a pre-anesthetic mix of Telazol (tiletamine/zolazepam)/Xylazine 3.5-5.5 mg/kg intramuscularly, endotracheally intubated and anesthetized with 3% inhalational isoflurane (with concentration adjusted as needed to maintain anesthesia). No paralytics were used during any part of the study. Normal saline was instilled at a maintenance rate (2 cc/kg/hr) via ear vein. Continuous cardiac monitoring was performed with a 3-lead EKG rhythm recorder
In each subject, a right lateral thoracotomy was performed to expose a region of esophagus. A series of 6 to 10 ablations, based on esophagus length, were placed directly on the esophagus using a 4mm ablation catheter (Safire 7Fr Quadripolar Catheter, St. Jude Medical, St. Paul, MN) powered by an RF generator (IBI 1500T9 RF, Irvine Biosciences Inc., Irvine, CA). RF energy was delivered via power control mode, holding wattage constant. Room-temperature saline was added to the thoracic cavity prior to performing ablations. Ablation energy was 10W with a 30-45s duration for all but one lesion, where 20W was utilized. The use of 10W represents an equivalent of approximately 30 to 40 Watts if the additional tissue of atrial wall, pericardium, adipose tissue, etc., was present and the ablation was being performed on the atrial wall and thermal impact measured on the esophageal mucosa. Contact force was measured on the first lesion to gauge pressure requirements, with subsequent lesions performed manually, matching the same level of force by an experienced electrophysiologist physician to achieve 15 g, as the catheter utilized for this study was not a contact-force measuring catheter. Since non-irrigated and non-contact-force sensing catheters are still used by 30% of the writing group of the 2017 HRS-EHRA-ECAS-APHRS-SOLAECE Expert Consensus Statement on Catheter and Surgical Ablation of Atrial Fibrillation, this first study serves as a reasonable starting point from which to advance knowledge in this important area of patient care.[21]
The esophageal heat transfer device was placed following standard procedure. Briefly, the device was connected to an external heat exchange unit (either a Medi-Therm III, Stryker Corp., Kalamazoo, MI, or a Thermotek Harmony, Thermotek Inc., Flower Mound, TX, supplying a minimum of 60 L/hour flow rate of water) according to standard procedures, both of which circulate distilled water as the coolant at a temperature range from 4ºC to 42ºC. After water flow was initiated, the tip of the device was lubricated with a water-soluble lubricant and inserted through the oropharynx into the esophagus to a depth sufficient for the tip to rest beyond the thoracic esophagus (See [Figure 1] for image of the esophageal device). The esophageal heat transfer device is a closed-loop cooling system, in that water does not leave the device but instead circulates in a closed-loop, counter-current configuration. The entire system is designed to utilize servo-mode cooling, which can also be considered a closed-loop feedback system; however, for this application, this feature was not necessary, as water temperature was pre-specified and set manually by the operators. Because the esophagus is a flat structure, compressed in the anterior-posterior direction when the patient is supine, firm contact with the anterior aspect of the esophagus occurs once placed into the esophagus. The open central lumen further provides gastric decompression, preventing distention of the esophagus away from the walls of the device. Because the entire length of the device contains water flow, the full length of the esophagus is contacted and cooled equally. Placement in the experimental animals was confirmed by visualization of the device distending the esophagus during entry, and palpation of the device in place once settled. Control lesions were performed with 37°C water held still within the device. Treatment lesions were performed with cooled water (range 5°C-37°C) circulating within the device. Each subject received a combination of control and treatment lesions. The presence of mucosal lesions was evaluated visually after triphenyltetrazolium chloride (TTC) staining and thermal injury depth was measured by target tissue histology, performed by a DVM and Diplomate of the American College of Veterinary Pathologists. Descriptive statistics are reported with comparisons of means via independent sample t-tests.
Figure 1. Image of the esophageal heat transfer device.

A model was developed utilizing COMSOL Multiphysics software (COMSOL, Burlington, MA, USA), utilizing similar methodology as found in others’ work in this area.[22,9,23] Mathematical modeling offers a powerful tool for predicting and confirming the dimensions and characteristics of lesions created under different ablation conditions, and allows evaluation of the impact of different parameters without requiring living tissue for each change in operating parameters of interest.[22] As such, we designed a model based on existing tissue parameters which we could then use to compare the results of the experimental data, and further serve as the basis for modelling of different ablation conditions using esophageal cooling for tissue protection. The 3D computational domain and subdomains were specified as shown in [Figure 2] The RF power was modeled using the Electric Currents interface from the ACDC module included in COMSOL Multiphysics. At the catheter tip boundaries, power (10W) and impedance (from 45Ω to 87Ω) were specified, based on the experimental settings and tissue response. The bottom face of the computational domain was set to ground. The heat transfer was modeled using the Bioheat Transfer interface from the Heat Transfer module included in COMSOL Multiphysics. The heat generation term was defined to be that produced by the RF power, using the coupling interface Electromagnetic Heat Source included in the Multiphysics module. Domain 5, the saline bath, was considered as a non-moving fluid. Further details are included in Supplementary Appendix 1.
Figure 2. Computational domain used for the mathematical modeling, with the ablation catheter applied directly to the esophagus, and with the cooling device inserted, and with subdomains including: 1) thoracic cavity (average tissue values), 2) saline water, 3) catheter body, 4) catheter tip, 5) esophagus, 6) cooling device

A total of 52 ablations were performed across 6 swine (average mass 81.5 ± 7kg). Six (6) ablations were used to determine experimental parameters. A total of 46 ablations were included for analysis, 23 treatment and 23 control. [Table 1] provides details on all lesions.
All ablations performed under control conditions produced external esophageal lesions; [Figure 3] shows external (adventitial) esophageal surfaces in-vivo, and [Figure 4] (top image) shows ex-vivo, while [Figure 4] (bottom image) shows a representative esophageal mucosal surface. Transmural lesions extending into the esophageal mucosa were consistently visible on gross examination after 30 seconds of 10W RF energy application in subjects less than 80kg. In subjects greater than 80kg, transmural lesions were obtained in at least 20% of cases with 30 seconds duration and 70% of cases with a 45 seconds duration.
In contrast, ablations performed under treatment conditions using 10W of RF energy using 37°C, 10°C, or 5°C circulating water, for 30 or 45s duration, did not produce visible transmural lesions and only 6 ablations (25%) produced visible external lesions. Ablations performed during the most aggressive treatment condition (5°C circulating water), did not demonstrate any visible lesions throughout the thickness of the esophageal musculature, including on the external surface of the esophagus at the point of contact with the ablation catheter.
Histopathological evaluation was performed with the measurement method as shown in [Figure 5], in which the maximum lesion thickness was determined, and divided by the maximum tissue thickness.
Measurements of lesion thickness confirmed that the percent transmurality of lesions decreased as water temperature flowing through the esophageal heat transfer device was decreased ([Table 1]). [Figure 6] shows a graphical representation of results. The absolute reduction in percent transmurality from control (45 seconds of application) with the use of 37°C water was 16.0% (p=0.2), while the absolute reduction with the use of 30°C water was 33.6% (p=0.02) and the absolute reduction using 5°C water was 35.6% (p=0.02). In the group with 30 seconds of RF application time, the absolute reduction in percent transmurality from control with the use of 37°C water was 5.1% (p=0.7), while the absolute reduction with the use of 10°C water was 69.7% (p<0.001) and the absolute reduction using 5°C water was 44.5% (p<0.001). Mean submucosal edema scores, muscularis mucosa damage scores, and epithelial damage scores likewise decreased with decreases in coolant temperature (and hence increases in heat extraction capacity).
Results of the model output, using tissue parameters as included in the COMSOL Multiphysics software and detailed in the Supplementary Appendix 1, revealed a close correlation to experimental findings, with an R^2 of 0.75. [Figure 6] shows the comparison between experimental and computational data.
Figure 3. In-vivo ablation application of the device and RF ablation directly on exposed esophagus (shown with normal saline water bath removed for clarity).

Figure 4. Top image: Sectioned esophagus showing application regions on external surface (lesions visible where no cooling applied, on left half of section). No lesions are visible on surface where cooling was applied with the device (right half of section). Bottom image: Sectioned esophagus showing mucosal surface (transmural lesions visible where no cooling applied).
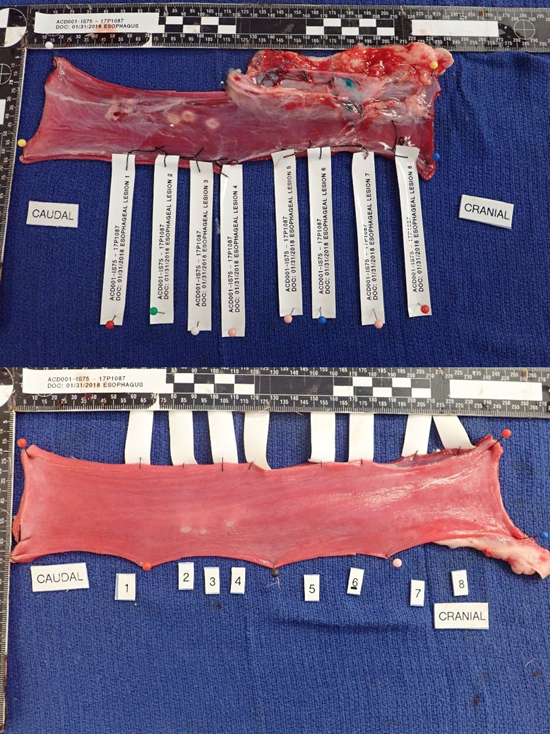
Figure 5. Max Tissue Thickness (in blue) included the entire thickness of the tissue on the slide at the site of measurement. Max Lesion Thickness (in black) included the thickness of the lesion starting at the adventitial connective tissue and going toward the epithelium to the maximum depth of the lesion damage. Measurement lines are illustrated separately for visual clarity but were taken at the same location for data collection to ensure accurate measurements for percentage calculation.
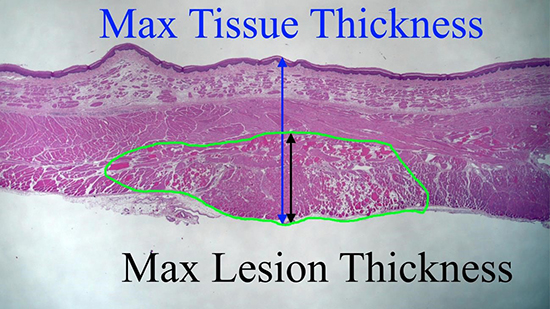
Figure 6 Percent lesion depth (transmurality) for each group of operational parameters, in experimental and modeling conditions.
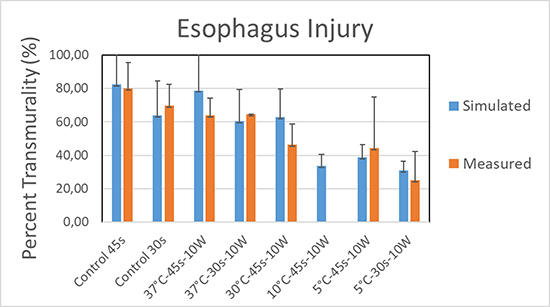
Table 1. Histopathology findings summarized by experimental group.
Group |
Number of lesions |
Energy (W) |
Duration (seconds) |
Cooling (yes/no) |
Temperature (degrees C) |
Percent transmurality (%) |
Std Dev (+/- %) |
Significance (P value) |
Mean submucosal edema score |
Mean muscularis mucosa damage score |
Mean epithelial damage score |
Control (45s) (n=8) |
8 |
10 |
45 |
no |
n/a |
79.9 |
15.7 |
Ref (45 sec) |
3.4 |
1.3 |
1.1 |
0.4 |
Control (30s) (n=15) |
15 |
10 |
30 |
no |
na |
69.7 |
12.6 |
Ref (30 sec) |
2.9 |
0.7 |
0.3 |
0.1 |
37C (45s) 10W (n=2) |
2 |
10 |
45 |
yes |
37 |
64.0 |
10.3 |
0.20 |
2.8 |
0.0 |
0.0 |
0.0 |
37C (30s) 10W (n=1) |
1 |
10 |
30 |
yes |
37 |
64.6 |
n/a |
0.70 |
0.3 |
0.0 |
0.0 |
0.0 |
30C (45s) 10W (n=2) |
2 |
10 |
45 |
yes |
30 |
46.3 |
12.3 |
0.02 |
1.5 |
0.0 |
0.0 |
0.0 |
10C (45s) 10W (n=2) |
2 |
10 |
45 |
yes |
10 |
0.0 |
n/a |
<0.001 |
0.0 |
0.0 |
0.0 |
0.0 |
5C (45s) 10W (n=4) |
4 |
10 |
45 |
yes |
5 |
44.4 |
30.4 |
0.02 |
0.8 |
0.0 |
0.0 |
0.0 |
5C (30s) 10W (n=12) |
12 |
10 |
30 |
yes |
5 |
25.2 |
17.1 |
<0.001 |
0.7 |
0.0 |
0.0 |
0.0 |
Score = (0) = None; Score = (1) = Minimal; Score = (2) = Mild; Score = (3) = Moderate; Score = (4) = Severe
Table 2. Summary of prior studies of esophageal cooling during RF ablation.
Modality/Device |
Study Method |
Flow Rate |
Temperature |
Results |
Citation |
Cooled intra-esophageal balloon |
Finite-element mathematical model |
N/A |
32, 25, and 15 C |
Chilling the esophagus minimizes the lesion in the esophageal wall |
[9] |
Intraesophageal balloon |
8 patient clinical study |
25 mL/min |
4.5 ± 3.1 C |
The intraesophageal balloon successfully lowers luminal esophageal temperature, and might prevent esophageal injury |
[13] |
Saline filled esophageal balloon |
Animal model |
Non-flowing |
10 C |
System was not sufficient to prevent thermal injury |
[14] |
Cooled intra-esophageal balloon |
Agar phantom |
25 mL/min |
5 C |
A cooled intraesophageal balloon provides effective thermal protection of the esophageal lumen |
[10] |
Cooled intra-esophageal balloon |
Agar phantom |
25 mL/min |
37, 23, 15, and 5 C |
A cooling balloon gives thermal protection to the esophagus with a minimum pre-cooling period of 2 min and a coolant temperature of 5 C or less |
[11] |
12 Fr probe with a distal expandable compliant latex sac (up to 3 cm in diameter) |
In-vitro and in-vivo animal model |
50 to 300 mL/ min |
25, 15, 10, and 5 C |
Device spares the esophagus from collateral thermal injury with circulating fluid at 5 or 10 C |
[12] |
Free water instillation |
100 patient clinical study |
5 mL aliquots |
Ice water |
Alleviated the severity of esophageal lesions, but did not significantly reduce the incidence |
[15] |
Free water instillation |
318 patient clinical study |
10 to 20 mL aliquots |
Ice water |
Esophageal damage reduced by infusing cooling solution into the esophagus |
[16] |
These data suggest a significant protective capability of a new esophageal heat transfer device against esophageal injury from the application of RF energy for ablation, with an accompanying mathematical model of the process developed to allow further investigation into this approach. Using a water temperature of 5°C supplied to an esophageal heat transfer device by either of two models of heat exchanger, a direct application of RF energy at 10 W for 30 seconds was unable to elicit visual evidence of thermal impact. In contrast, under control conditions without water flow through the device, this same energy resulted in fully transmural lesions visible on gross pathology. Histologic analysis demonstrated a marked reduction in transmurality of lesions with use of the device, and mean submucosal edema scores, muscularis mucosa damage scores, and epithelial damage scores were likewise notably reduced. Even at a coolant temperature of 37°C, a protective effect was seen, suggesting that the high coolant flow rates obtained with the external heat exchange units (minimum of 60 L/hour) may be an important component of this effect. In comparison, earlier studies of esophageal cooling for thermal protection found generally that efficacy appeared to increase with increasing flow rates, but the range utilized in these earlier studies was typically 25 mL/minute to at most 300 mL/minute, much less than the 1000 mL/minute minimum used in this current study. [Table 2] summarizes these earlier studies
Collectively, these data from prior investigations into esophageal protection via direct cooling suggest that although some efficacy was apparent, a limitation stemmed from the lower, or absent, flow rates of coolant employed. Additionally, it was noted in one paper that the methods previously investigated were somewhat complicated to perform in clinical practice, and thus no follow-up studies were conducted.[15] In contrast, the esophageal heat transfer device evaluated in this study is straightforward to deploy without disruption in typical workflow in the electrophysiology lab. This study did not investigate the potential to protect against injury from cryoablation; however the device is also capable of warming the esophagus.
A number of clinical studies of this approach are underway, utilizing the device investigated in this study. These include: Improving Oesophageal Protection During AF Ablation (IMPACT) - NCT03819946, Esophageal Cooling for AF Ablation (eCoolAF) - NCT03691571, and Esophageal Damage Protection During Pulmonary Vein Ablation. Pilot Study. - NCT03832959. In addition, initial clinical data from a single site using a milder setting of 30°C water temperature have been presented.[24]
This study did not utilize a contact-force sensing catheter to measure applied force at each lesion. The first application utilized force measurement with an external gauge, while all subsequent lesions relied on the judgement of an experienced electrophysiologist. Although some variability in contact force is inevitable with this approach, the force was applied directly, without having to navigate through a percutaneous approach with the associated variable resistances through vasculature that would add more variability to the contact force applied. Moreover, a systematic bias is unlikely, and the effect size seen in this approach would likely overwhelm the variability in contact force. Likewise, in lieu of irrigation supplied through the tip of the ablation catheter, saline was used as a water bath during ablations; however, this likely provides a more severe thermal insult to the tissue than would be the case with irrigation. This study did not involve ablation of the atria directly; however, the design that was utilized (ablating directly on the adventitial surface of the esophagus) provided a worst-case model that eliminates confounders such as variations between subjects in location of the esophagus relative to the atria, variations in the amount of interspersed tissue, and variations in atrial wall thickness, all of which would confound the data. An energy level of 10 W was chosen, since the ablations were performed directly on the esophagus. This is equivalent to higher wattages applied to the atrial wall by a factor of 3-4x (due to the significantly greater amount of tissue through which the energy must traverse). Additionally, this study does not address what impact the esophageal heat transfer device may have on the atrial tissue, but the much higher flow rate of blood through the atria is likely to overwhelm the cooling effect such that no significant temperature reduction of the atrial tissue occurs. Mathematical modeling of this is currently underway and further supports this concept. No lesions were identifiable in the region of RF ablations during cooling with 10°C water flow and 30 seconds of duration using 10W of power, which may reflect inadvertent misplacement of sectioning knife for tissue histology, variation in performance of the device, or variation in contact force applied. Finally, protection against AEF using this approach is predicated on the hypothesis that visible lesions on the esophageal mucosa are a precursor to AEF development, Although this hypothesis is generally accepted, additional mechanisms such as delayed ischemic necrosis may potentially play a role in progression to AEF.
Use of a new esophageal heat transfer device resulted in significant protection against esophageal injury from direct radiofrequency ablation. The protective effects seen in these data suggest that this may be an effective approach to the prevention of esophageal injury during RF ablation involving the posterior wall of the left atrium. A mathematical model of the process provides a means to further evaluate and refine this approach to preventing esophageal injury during RF ablation and can serve to guide ongoing clinical investigations currently in progress.